The International Thermonuclear Experimental Reactor (ITER) project, a global collaboration aimed at harnessing nuclear fusion, achieved a monumental engineering feat in August 2024: the completion of its first vacuum vessel sector, weighing an astounding 5,200 tons. This accomplishment is not only a significant milestone for ITER but also a major leap forward in the pursuit of sustainable, clean energy through nuclear fusion. ITER’s vacuum vessel is one of the most critical components in the experiment, designed to confine the plasma in which fusion reactions occur. The completion of the first sector marks a pivotal moment in the long journey toward producing unlimited energy, mimicking the process that powers the sun.
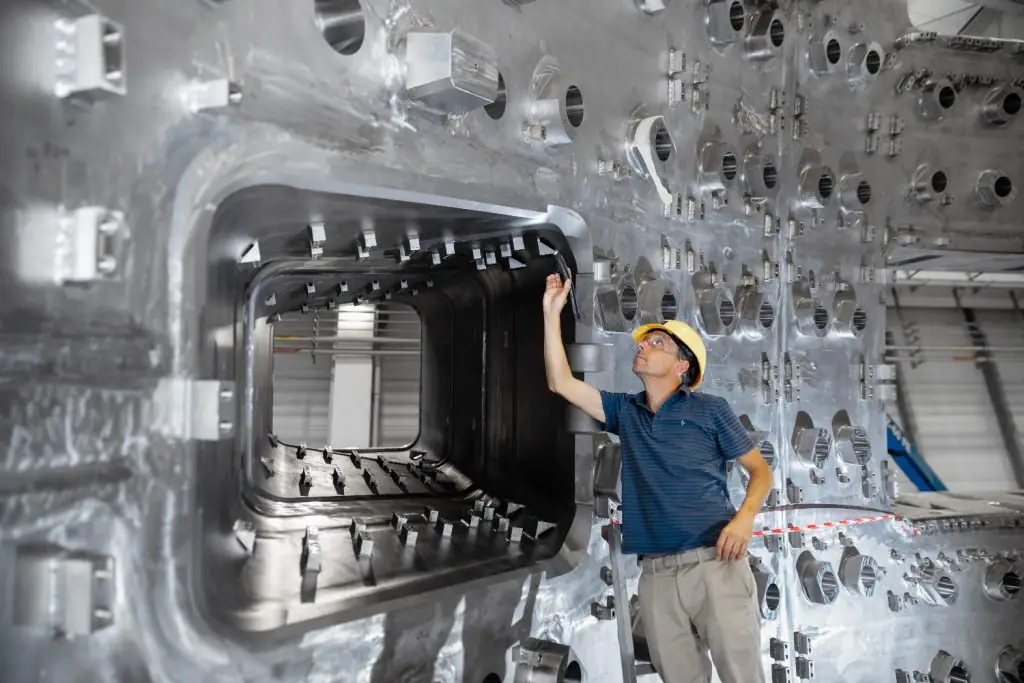
The Importance of the Vacuum Vessel
The vacuum vessel is at the heart of the ITER reactor. It is a double-walled, hermetically sealed, stainless steel container that plays a crucial role in plasma confinement, radiation shielding, and structural support for the reactor. Measuring 19.4 meters in diameter, 11.4 meters in height, and weighing approximately 5,200 tons, the ITER vacuum vessel is one of the largest and most complex pieces of equipment ever built for a fusion reactor.
One of the primary functions of the vacuum vessel is to create a high-vacuum environment necessary to maintain plasma stability. In fusion reactions, plasma—an extremely hot, ionized gas made of charged particles—must be contained and prevented from coming into contact with the reactor walls. The temperature of the plasma inside the vessel reaches as high as 150 million degrees Celsius, far exceeding that of the sun. This necessitates the use of superconducting magnets, placed outside the vessel, to generate powerful magnetic fields that confine the plasma in a doughnut-shaped structure, or “tokamak.” This magnetic confinement ensures that the plasma remains suspended, preventing any material damage to the reactor’s components.

Additionally, the vacuum vessel acts as the primary barrier to radioactivity, ensuring that the fusion reactions inside are securely contained. The vessel’s design includes space for internal components such as the blanket and the divertor, which contribute to radiation shielding and energy extraction. The blanket modules, which line the inside of the vacuum vessel, absorb the neutrons produced during fusion, converting their kinetic energy into heat. Some of these blanket modules will also test new materials for breeding tritium—a necessary fuel for sustaining future fusion reactions.

Engineering Feats and Global Collaboration
Building the vacuum vessel is a massive undertaking that involves a complex international supply chain. ITER is a collaboration of 35 nations, including the European Union, the United States, China, India, Japan, Korea, and Russia. Europe is responsible for building five of the vacuum vessel’s nine sectors, while Korea is building the remaining four. The completed vacuum vessel will consist of these nine individual sectors that, when assembled, will create a structure weighing over 8,500 tons.
The construction of each vacuum vessel sector is an extraordinary engineering challenge. The first completed sector, known as sector 5, was manufactured by a European consortium composed of Ansaldo Nucleare, Westinghouse, and Walter Tosto, under the supervision of Fusion for Energy (F4E)—the European Union’s agency managing its contributions to ITER. The production process took several years to complete and involved over 150 kilometers of welding. Each weld had to meet stringent safety and quality standards, as the French Nuclear Safety Authority regulates the project.
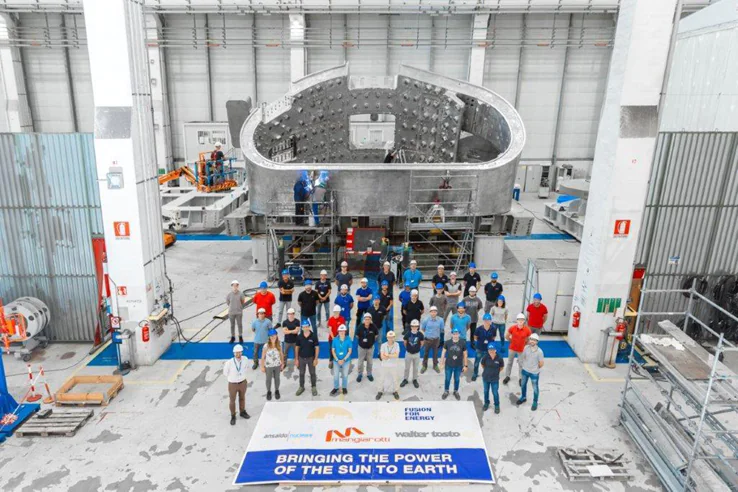
One of the unique challenges of constructing the vacuum vessel is ensuring that each sector can withstand the extreme operational conditions inside the reactor, including massive temperature fluctuations, intense radiation, and strong electromagnetic forces. Once all nine sectors are delivered and installed at the ITER site in southern France, they will be welded together inside the reactor pit. This will be one of the largest industrial assembly projects ever attempted in nuclear engineering, requiring precision down to the millimeter.
Vacuum Vessel in Action: How It Will Sustain Fusion
ITER’s vacuum vessel is designed to be the most advanced tokamak ever built, offering an unprecedented volume of 1,400 cubic meters for plasma confinement. The size of the vessel is crucial because it allows for the sustained confinement of high-energy plasma, increasing the likelihood of achieving a self-sustaining fusion reaction—also known as “ignition.” The larger the volume, the longer the plasma can be confined, which in turn enhances the probability of maintaining the high temperatures and pressures needed for fusion.
Fusion is the process by which two light atomic nuclei, typically isotopes of hydrogen, fuse together to form a heavier nucleus, releasing vast amounts of energy. In the case of ITER, the fusion fuel will consist of deuterium and tritium, both isotopes of hydrogen. When these nuclei collide at extremely high speeds, they overcome their natural repulsion and fuse together, releasing a neutron and a helium nucleus. The released energy comes from the difference in mass between the reactants and the products, according to Einstein’s equation E=mc2E = mc^2E=mc2.
The neutrons produced in the fusion reaction are absorbed by the vacuum vessel’s blanket modules, which serve multiple functions. In addition to shielding the reactor from neutron radiation, these modules are designed to capture the energy of the neutrons and convert it into heat. This heat can then be used to produce electricity, making fusion a potentially unlimited and clean source of energy.
Another key function of the vacuum vessel is to provide support for the reactor’s divertor, a component that extracts impurities from the plasma and helps maintain its stability. The divertor, located at the bottom of the vacuum vessel, plays a critical role in removing helium “ash”—the byproduct of the fusion reaction—which could otherwise cool down the plasma and halt the fusion process.
Looking Ahead: The Path to Sustainable Fusion Energy
The completion of the first vacuum vessel sector marks a significant step forward in ITER’s journey toward producing fusion energy. Once all nine sectors are installed and the reactor becomes operational, ITER will be the first fusion experiment to produce more energy than it consumes. It aims to demonstrate that fusion can be a viable, large-scale, and carbon-free energy source for the future.
However, there is still much work to be done before ITER achieves its ultimate goal of sustained fusion. The construction of the vacuum vessel is just one of many challenges that the project faces, but its completion brings scientists closer to the dream of harnessing the power of the stars to meet the world’s growing energy demands. When fully operational, ITER will pave the way for the development of commercial fusion power plants, which could revolutionize the global energy landscape by providing a virtually limitless, clean, and safe energy source.
In summary, the ITER vacuum vessel is a crucial component in the quest for nuclear fusion. Its successful construction marks a significant engineering achievement, made possible by international collaboration and cutting-edge technology. As the ITER project progresses, the vacuum vessel will play an essential role in enabling sustainable fusion energy, offering the promise of a cleaner, more abundant energy future for the world.